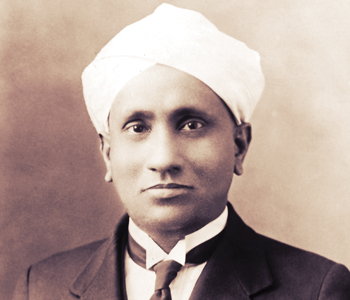
C.V. Raman
Chandrasekhara Venkata Raman was born in 1888 in a village in southern India. As a child, Raman was precocious, curious and highly intelligent. His father was a college lecturer in mathematics, physics and physical geography, so the young Raman had immediate access to a wealth of scientific volumes. By the age of 13, he had read Helmholtz’s Popular Lectures on Scientific Subjects.
Raman was deeply interested in music and acoustics. While in college, he read the scientific papers of Lord Rayleigh and his treatise on sound as well as the English translation of Helmholtz’s The Sensations of Tone. This initiated Raman’s later interest in the physics of drums and stringed instruments such as the violin. He used fine-chalk powder and photography to investigate the vibrational nodes of drums; the white chalk remained only at the nodes of the vibrating membrane.
In a culturally anomolous and brazen act, when Raman was 18, he arranged his own marriage to Lokasundari (later called Lady Raman), a 13-year-old woman from Madras. The two then moved to Calcutta, where Raman accepted a position in the Indian Finance Department. During the next ten years—from 1907 to 1917—he struggled to balance his well-paying government job with his drive to be a scientist.
When he wasn’t at the Finance Department, he was conducting experiments at the Indian Association for the Cultivation of Sciences (IACS) in Calcutta. The IACS had been formed along the pattern of the Royal Institution in London. Its journal Proceedings was renamed the Indian Journal of Physics in 1926. Raman’s early works become known to an international audience when he published his research in the journals Nature, Philosophical Magazine and the Physical Review.
By 1917, Raman had had enough of his double life. He quit his government position and devoted himself fully to science. He accepted a full-time professorship—the endowed Pailt Chair of Physics—at Calcutta University, where he remained for 15 years.
One of the requirements of that position was to obtain training abroad in order to achieve parity with foreign professionals. Confident in his genius, Raman claimed that he did not need any foreign training; on the contrary, he was prepared to train those from other countries. Moreover, he argued, he had already earned a prestigious international reputation in physics due to his publications. Since Raman refused to budge, the University had no choice but to waive this requirement in order to secure the rising star. In 1924, Raman was elected a Fellow of the Royal Society. It is as if he knew he was destined for greatness. Indeed, in 1925, when Raman was attempting to obtain funds to purchase a spectroscope, he told his benefactor: “If I have it, I think I can get a Nobel Prize for India.”
In 1933, Raman became director and professor at the Indian Institute of Science (IIS) at Bangalore. The next year, he established the Indian Academy of Sciences. Over the following decade, he published more than 30 papers in the Proceedings of the Indian Association for the Cultivation of Science, Nature, Philosophical Magazine and Physical Review. In 1937, he quit his position following disputes with some staff and members of the Council of the IIS.
At the age of 60, Raman then formed the Raman Research Institute (supported with his own funds and donations that he raised). He also remained a professor, as well as the President of the Indian Academy of Sciences in Bangalore, until his death in 1970.
How did Raman discover the Raman effect?
In 1921, Raman had traveled to Europe from his home in Calcutta to attend the Congress of Universities of the British Empire at Oxford. While he was there, he conducted some acoustic research on the central gallery of St. Paul’s Cathedral in London. He also met with three outstanding British physicists: Joseph J. Thompson, Ernest Rutherford and William H. Bragg. He lectured to the Physical Society on his research in acoustics and optics.
But it was his trip home that would lead Raman to change history. During his sea voyage, he observed the blue opalescence of the Mediterranean and wondered about the origin of this beautiful phenomenon. Raman was aware of Lord Rayleigh’s explanation—that the color of the sea was due to the reflection of the blue sky—but he did not accept it. So, with a polarizing Nicol quartz prism that he carried in his pocket, he proceeded to demonstrate Rayleigh’s explanation to be false; he quenched the surface reflection of the sky on the sea surface, and noted that the blue color of the sea was unattenuated. With a diffraction grating, he showed that the maximum spectral intensity was different for the blue sky and the blue sea.
During this voyage, Raman sent two papers to the journal Nature positing that the color of the sea was due to light scattering by the water molecules—a phenomenon he called molecular diffraction. Thus began Raman’s new research obsession: the molecular basis of light scattering.
Back home in India, Raman and his students observed the frequency shift of scattered light. They knew the phenomena was not Rayleigh scattering, since that type of scattering did not produce a frequency shift; however, they needed to exclude the possibility that minute traces of fluorescence were causing the shift. To do this, they purified the liquids multiple times. When the phenomenon remained, they concluded that it was not due to fluorescence.
By 1925, Raman had observed the frequency-shifted scattered light in more than 50 liquids and, by 1927, he had noticed that the scattered light was polarized. He described the phenomenon—which he called modified scattering—in a paper in Nature. Later it would be called the Raman effect.
In his explanation of the new phenomenon, Raman showed that the frequency shift is a characteristic of the molecule comprising the scattering medium; it is independent of the frequency of the incident light. This differentiated the Raman effect from fluorescence, which strongly depends on the frequency of the incident light. There are notable exceptions, of course: Brillouin scattering and Raman scattering coupled to acoustic waves in a condensed medium (acoustic-optical effects in crystals). Raman spectra of molecules differ from infrared spectra in their selection rules and their polarization characteristics; however, the measured frequency shifts of the Raman lines correspond to the infrared frequencies of the scattering molecules.
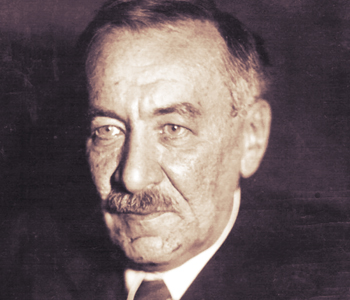
Leonid Mandelstam
Raman’s equipment and experimental set-up
The main challenge Raman faced in his experimental work was posed by the extremely weak intensity of the scattered light. In his early studies, Raman used a heliostat—a mechanically driven mirror that tracked the motion of the sun to provide a light source. Eventually, however, he came to realize that the sunlight was not sufficiently intense on its own. Thus, in 1927, he acquired a 7-in. refracting telescope, which he used in combination with a short-focus lens to condense the sunlight into a narrow beam.
The following year, he created an even more powerful light source by using highly monochromatic light from a mercury arc lamp together with a large-aperture condenser and cobalt-glass filter. Sometimes he replaced the glass filters with liquid ones.
Raman used a violet filter to isolate a band of violet light incident on a sample liquid. At 90 degrees to the incident light, he placed another violet glass filter. This enabled him to observe violet light scattered from the sample, which represented normal Rayleigh scattering. When he replaced the second filter with a green one, however, the Rayleigh-scattered light was blocked but there was still some green light visible, demonstrating a second form of scattering.
Perhaps most interestingly, Raman used his own dark-adapted eyes as photodetectors. Only after he had observed the frequency shift with his eyes and a direct-vision spectroscope did he repeat the observation with a mercury arc lamp and a Hilger baby quartz spectrograph. Surprising as it may seem, the human eye can detect single photons over a high dynamic range.
Raman used a small Adam Hilger spectroscope for his initial studies, and he detected the spectrum of the scattered light using photography. Since the intensity of the frequency-shifted light was extremely weak, long exposure times were required to record the spectra.
Attribution of credit
Raman was both a prolific investigator and a skilled communicator. By the late 1920s, he was achieving recognition for his work on the Raman effect—due in part to his tireless efforts to demonstrate and distribute his results. After his first publication of the Raman spectra in the March 16, 1928, Indian Journal of Physics, Raman mailed 2,000 reprints to scientists in the United States, Canada, France, Germany and Russia. In this way, Raman consolidated his priority and credit for the discovery. Shortly afterwards, the Raman effect was confirmed by some of the world’s most authoritative physicists in the field of light scattering and optics in France, Canada, Germany, the United States and Italy.
In 1929, the Faraday Society of London held a special symposium dedicated to the Raman effect. That same year, Raman was knighted by the British government in India. The following year, he was given the Hughes Medal by the Royal Society. Also in 1930, Raman received the Nobel Prize in Physics for his “‘investigations on the scattering of light and the effect named after him.”
Not everyone agreed that Raman deserved full credit for discovering the Raman effect. After all, Smekal had provided the theoretical basis for light scattering in 1923, and Landsberg and Mandelstam had simultaneously discovered the Raman effect on solid quartz in 1928. Why was the Nobel given only to Raman?
First, Smekal’s work was not widely known at the time that Raman had conducted his scattering experiments. A letter summarizing Smekal’s findings was published in Die Naturwissenschaften, but it was not abstracted and most likely had not been seen by Raman and his colleagues.
As for Landsberg and Mandelstam, they had published their results after Raman’s were in print. In addition, their paper cited previous works by Raman; although these corresponded to articles that had been published prior to Raman’s March 1928 Nature article detailing his discovery, these references perhaps confused the Nobel Committee and led them to believe that the Russians’ work did not represent an independent and simultaneous discovery.
Moreover, Landsberg and Mandelstam did not at first publish their results of scattering at a shifted frequency; instead they gave an oral presentation at a conference in Moscow in April 1928 based on their measurements, which were taken in February of that year. By the time they submitted their results in May 1928 and published them in July, 16 papers had been published on the Raman effect, many by Raman and his colleagues.
Still, many Austrian, German and Russian physicists felt strongly that credit should be shared. They refused to adopt the name “the Raman effect,” and referred instead to “combination scattering” or “the Smekal-Mandelstam-Raman scattering.” In 1931, K.W.F. Kohlrausch, an Austrian physicist, gave his book a title that recognized both Smekal and Raman: Der Smekal-Raman Effekt.
In fact, some of the Nobel nominations for the 1930 award included other scientists in recognition of the Raman effect. One nomination went jointly to Raman and Heisenberg, who further developed Smekal’s concepts and contributed to a quantum theory of dispersion by atoms. Two others recognized Raman and R.W. Wood, the American scientist who confirmed Raman’s experiments. Another was for Raman, Landsberg and Mandelstam.
But the Nobel Committee decided the award should go to Raman alone, and the rest is history. Raman is the only India Nobel laureate whose award in physics was based on work completed in India. He was a great man known for his driving ambition and passion for science. A few days before his death on November 21, 1970, Raman spoke these words, “Science can only flower out when there is an internal urge. It cannot thrive under external pressure.” A tree grows where Raman died.
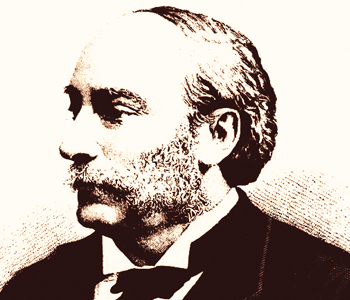
Lord Rayleigh
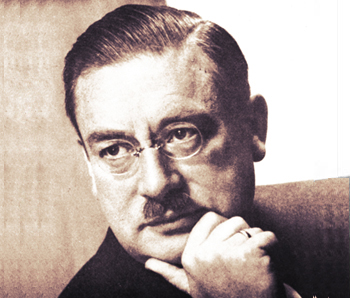
Peter Debye
Research on Light Scattering in the 1920s.
Light scattering was a popular research area in physics laboratories worldwide in the 1920s. The topic was under investigation by Lord Rayleigh in England, Jean Cabannes in Paris, Robert W. Wood in New York, and Grigory Landsberg and Leonid Mandelstam in the Institute of Physics in Moscow.
Following in the footsteps of Albert Einstein and Marian Smoluchowski, Mandelstam developed a theory of light scattering at an interface that varied by fluctuations. At the same time, Peter Debye published his theory about the specific heats of solids using concepts about propagating elastic waves in solids. By the time Mandelstam made the connection between the Fourier components in his theory and Debye’s elastic waves, it was too late; Leon Brillouin, working independently in France, had already published a theoretical paper explaining that scattered light could be shifted in frequency.
Let’s take a step back and explore elastic scattering. If the scattered photon has the same energy as the incident one, but a different direction of propagation, the result is elastic scattering. Examples include Rayleigh scattering (with particles much smaller than wavelength of light) or Mie scattering (particles of a size similar to the wavelength of light). In Rayleigh scattering, energy is conserved, and its intensity is proportional to the fourth power of the incident frequency. The oscillating electric field induces dipoles in the material that radiate the light; this occurs in the plane perpendicular to the dipole and also perpendicular to the electric field vector of the incident light.
In the much weaker processes of Raman and Brillouin scattering, however, the internal energy of the scatterer changes. Brillouin scattering refers to the transfer of energy to acoustic modes of vibration in the material; it differs from the optical modes that are excited in Raman scattering. These are inelastic scattering processes, in which the photon’s energy and frequency are changed. A photon is absorbed, raising the molecule to a higher energy state; then a photon is emitted and the molecule moves into a different energy state (vibrational or rotational) from the initial one.
The Stokes and the anti-Stokes lines are equally displaced from the Rayleigh line; the Stokes line has the higher intensity. In condensed matter, Raman scattering is described quantum mechanically by the exchange of a phonon (quanta of mechanical energy) between a photon and the non-propagating modes of excitation of the condensed matter.
The Austrian physicist Adolf Smekal provided the theoretical basis for inelastic light scattering in 1923. This type of scattering was also implied in the dispersion theory of Hendrik A. Kramers and Werner Heisenberg (1925). Smekal proposed that photons could be scattered inelastically by vibrational transitions of molecules (Die Naturwissenschaften, 11, 1923). He assumed the quantum nature of light, used Bohr’s Correspondence Principle, and predicted that the scattered monochromatic light would consist of the original wavelength together with longer and shorter wavelengths. Smekal showed that the shift in frequency between the incident and scattered light corresponds to the energy difference between two states of the molecule.
Kramers and Heisenberg further developed Smekal’s concepts and published their quantum theory of the dispersion by atoms in 1925. They showed that the frequency-shifted light was incoherent, and they introduced the concept of a “virtual state.” Later, they realized that Smekal’s note contained an important concept: The Raman effect corresponded to a transition between two discrete levels and all forms of excitation. Subsequently, many types of Raman effects were observed in solids.
In their 1925 paper, Kramers and Heisenberg used the Bohr correspondence principle and extended Smekal’s previous work on incoherent scattering. They stated the possibility of the converse process: An atom in an excited state collides with a photon, and, following the collision, the atom shifts to the lower energy state, while the scattered photon is shifted in frequency to higher energy; i.e., a red incident light is scattered as a blue light. The scientists postulated that irradiating an atom with monochromatic light results in the atom radiating coherent spherical waves (Rayleigh scattering) and also incoherent spherical waves (Raman scattering) whose frequencies are combinations of the incident frequency and frequencies that correspond to possible transitions to other stationary states.
Meanwhile, Landsberg and Mandelstam were studying the theories of the specific heats of solids and the published works of Einstein and Debye. They investigated Brillouin scattering from large samples of quartz. Their light source was a mercury arc lamp with a filter to narrow the bandwidth of the excitation light. They placed a spectrograph at 90 degrees to the incident light. While the scattering effect from liquids was strong, the similar effect from quartz was extremely weak and necessitated a 15 hour exposure time. These Russian physicists independently rediscovered the Raman effect in crystalline quartz and calcite; their work was published in 1928.
Barry R. Masters OSA Fellow, SPIE Fellow, is with the department of biological engineering, MIT, Cambridge, Mass., U.S.A.
References and Resources
- Lord Rayleigh, Colours of the Sea and Sky, Nature 83, 48ff (1910).
- A. Smekal. “On the quantum theory of dispersion,” Die Naturwissenschaften, 11, 873-875 (1923).
- H.A. Kramers and W. Heisenberg. “On the dispersion of radiation by atoms,” Zeitschrift für Physik, 31, 681-708 (1925).
- R.W. Wood. “Wavelength shifts in scattered light,” Nature 122, 349, (1928).
- K.W.F. Kohlrausch. Der Smekal-Raman-Effect, Berlin: Verlag Von Julius Springer, (1931).
- R.S. Krishnan and R.K. Shankar, “Raman effect: history of the discovery,” J. Raman Spectrosc. 10, 1-8 (1981).
- G. Venkataraman, Journey into Light, Life and Science of C. V. Raman, Indian Academy of Sciences in co-operation with Indian National Science Academy, Bangalore, India (1988).
- C.V. Raman, Scientific Papers of C. V. Raman, Six Volumes, edited by S. Ramaseshan, Bangalore, India: Indian Academy of Sciences, (1988). Vol 1. The Scattering of Light, Vol. 2. Acoustics, Vol. 3. Optics, Vol. 4. Optics of Minerals and Diamonds, Vol. 5. Physics of Crystals, Vol. 6. Floral Colours and Visual Perception.
- C.V. Raman, Scientific Papers, The Collected Papers of C. V. Raman are available at The Raman Research Institute, Digital Repository
- S. Ramaseshan and C.R. Rao, C. V. Raman, A Pictorial Biography, Bangalore, India: Published by The Indian Academy of Sciences (1988).
- Indian Academy of Sciences, Bangalore, India
- Raman Research Institute, Bangalore, India
- C.V. Raman, The Molecular Scattering of Light, Nobel Lecture in Physics, December 11, 1930